Super-resolution velocity and distance estimation method based on kernel density
Limitations of Conventional Doppler Analysis
Pulsed Doppler radar can be used to estimate Doppler velocity to improve distance resolution and to identify human bodies. The Fourier transform-based method is a common method for Doppler velocity estimation, but it requires a high center frequency and long observation time to achieve high Doppler velocity resolution. However, a wall-transmitting UWB radar, which requires penetration through dielectric materials, requires a low center frequency. In addition, when the super-resolution distance estimation method is applied, the output response is almost impulsive, so the carrier frequency is lost and processes such as the Fourier transform, which measures the amount of phase rotation described above, cannot be applied. Furthermore, the above impulsive response and the UWB signal have a very wide specific bandwidth, and the time that the reflected pulses exist in the same range gate (effective observation time) becomes short. Therefore, it is difficult to maintain high velocity resolution in the wall transmission model with Doppler velocity estimation based on the Fourier transform.
Super-resolution Doppler analysis method based on kernel density estimation
To solve the above problem, we have proposed a completely new Doppler analysis method based on kernel density estimation. This method calculates the slope of the observed arrival distance in the time direction and estimates their distribution as a statistical distribution with a Gaussian kernel, thereby enabling highly accurate estimation of the Doppler velocity at each distance and time. Figure 1 shows the performance evaluation based on a simulation of a walking human body. Conventional Fourier transform methods cannot extract the true velocity information due to insufficient velocity resolution. In contrast, the combined use of a compressed sensing filter and the kernel density estimation method allows the velocity of each part of the human body to be estimated at each time point. In principle, this method does not use coherent processing, so it can achieve high Doppler velocity resolution with very short time resolution. Furthermore, by integrating this method with the RPM method, the Doppler velocity can be linked upward to the image, as shown in Figure 2, to achieve higher-order image analysis and identification. Currently, these methods are being evaluated in a real environment and on a real human body, and research is underway for their practical application.
[1]Takeru Ando and Shouhei Kidera, "k- and Doppler Velocity Decomposition Based Range Points Migration for Three-dimensional Localization with Millimeter Wave Radar",
IEEE Sensors Journal, (in press) 2022.
[2]Takumi Hayashi, Takeru Ando, and Shouhei Kidera "Accurate Doppler Velocity Estimation by Iterative WKD Algorithm for Pulse-Doppler Radar", , IEICE Trans. Commun., (in press), 2022.
[3]Takeru Ando and Shouhei Kidera, "Accurate Micro-Doppler Analysis by Doppler and k-space Decomposition for Millimeter Wave Radar", IEEE Journal of Selected Topics in Applied Earth Observations and Remote Sensing, 2022.
[4]Y. Akiyama, T. Omori, and S. Kidera, " k-Space Decomposition Based Three-dimensional Imaging with Range Points Migration for Millimeter Wave Radar", IEEE Trans. Geoscience & Remote Sensing,vol. 59, no. 8, pp. 6637-6650, Aug. 2021.
[5]M. Setsu, T. Hayashi, J. He, and S. Kidera, " Super-Resolution Doppler Velocity Estimation by Kernel-Based Range-Ą Point Conversions for UWB Short-Range Radars ", IEEE Trans. Geoscience & Remote Sensing, Vol. 59, 2020.
Pulsed Doppler radar can be used to estimate Doppler velocity to improve distance resolution and to identify human bodies. The Fourier transform-based method is a common method for Doppler velocity estimation, but it requires a high center frequency and long observation time to achieve high Doppler velocity resolution. However, a wall-transmitting UWB radar, which requires penetration through dielectric materials, requires a low center frequency. In addition, when the super-resolution distance estimation method is applied, the output response is almost impulsive, so the carrier frequency is lost and processes such as the Fourier transform, which measures the amount of phase rotation described above, cannot be applied. Furthermore, the above impulsive response and the UWB signal have a very wide specific bandwidth, and the time that the reflected pulses exist in the same range gate (effective observation time) becomes short. Therefore, it is difficult to maintain high velocity resolution in the wall transmission model with Doppler velocity estimation based on the Fourier transform.
Super-resolution Doppler analysis method based on kernel density estimation
To solve the above problem, we have proposed a completely new Doppler analysis method based on kernel density estimation. This method calculates the slope of the observed arrival distance in the time direction and estimates their distribution as a statistical distribution with a Gaussian kernel, thereby enabling highly accurate estimation of the Doppler velocity at each distance and time. Figure 1 shows the performance evaluation based on a simulation of a walking human body. Conventional Fourier transform methods cannot extract the true velocity information due to insufficient velocity resolution. In contrast, the combined use of a compressed sensing filter and the kernel density estimation method allows the velocity of each part of the human body to be estimated at each time point. In principle, this method does not use coherent processing, so it can achieve high Doppler velocity resolution with very short time resolution. Furthermore, by integrating this method with the RPM method, the Doppler velocity can be linked upward to the image, as shown in Figure 2, to achieve higher-order image analysis and identification. Currently, these methods are being evaluated in a real environment and on a real human body, and research is underway for their practical application.
Figure 1: Example of micro-Doppler analysis on a human body (left: observed model, center: conventional method, left: proposed method)
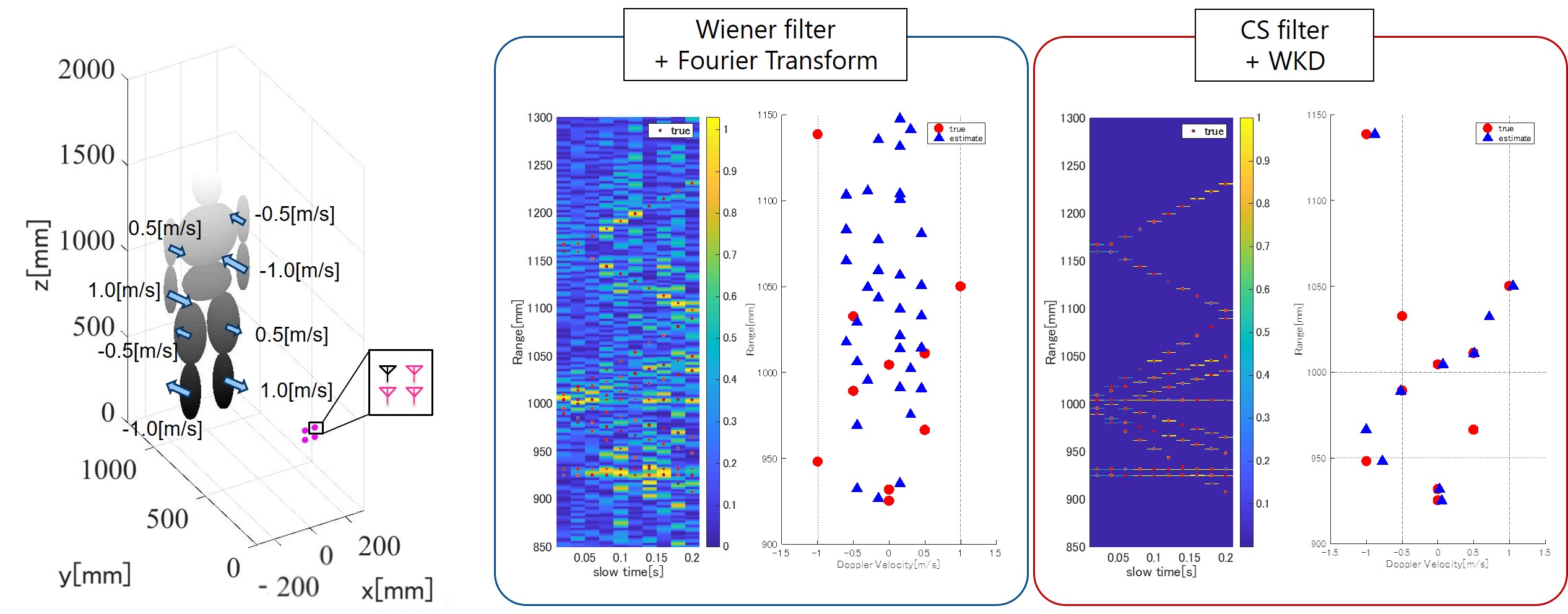
Figure 2: Observation model (left), example of imaging integrating RPM method and Doppler velocity
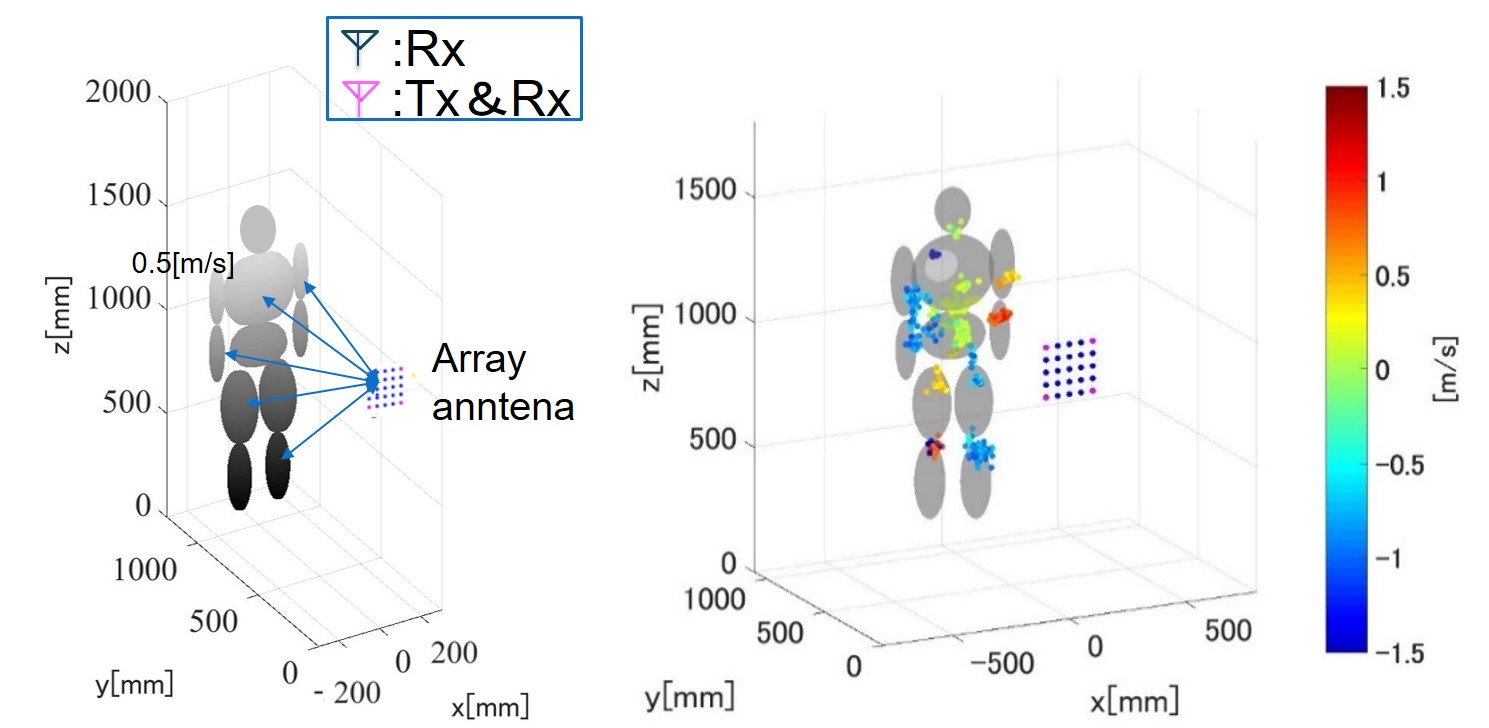